Key Takeaways
- VR optics utilize head-mounted displays (HMDs) to immerse users in virtual environments by projecting virtual scenes through an optical system, reducing eye strain.
- Key performance indicators include field of view (FOV) for immersion, EyeBox for comfortable eye movement, distortion control for image accuracy, and dispersion reduction to prevent color fringing.
- Optical solutions vary by design: aspherical lenses reduce spherical aberrations, Fresnel lenses are lightweight but can distort images, and Pancake lenses are compact and high-quality but more expensive.
An Introduction To VR Optics
A head-mounted display (HMD)—commonly referred to as a headset—projects signals from the display screen to the eyes through an optical system, creating a visual experience distinct from reality. Virtual Reality (VR) technology has rapidly advanced, immersing users in entirely virtual environments that feel like another world. At the core of VR display technology lies optical imaging, which projects virtual scenes to users’ eyes, enabling realistic visual experiences.
Without an optical system, displays placed too close to the eyes would require constant diopter adjustments, leading to eye strain or damage. Moreover, if the display is closer than the eye’s focal adjustment range, imaging becomes impossible. VR optical systems address this by converting the near-eye display’s point light signals into approximately parallel light rays, minimizing diopter adjustment strain and creating a comfortable, immersive experience.
Early VR optical systems used single lenses, borrowing principles from simple eyepieces like magnifying glasses. However, modern VR systems must balance imaging quality, device size, and weight, leading to the development of specialized optical solutions.
Indicators of VR optics
- Field of view
Field of view (FOV) is a critical metric that directly impacts user immersion. FOV refers to the maximum visible area users can see when wearing a headset, defined as the angle between the edges of the field of view. If expressed in the form of an angle, the size of the field of view is the size of the angle between the field of view at the edge of the object and the eye, that is, the maximum visible range, which is called the field of view angle.
The field of view angle is an important parameter to judge immersion, which defines the visible area that VR users can see when wearing a headset. A wider field of view allows for a more immersive experience, allowing users to truly be in a virtual environment. In VR optics, 90° FOV is considered to be the pass line for VR immersion experience, 120° FOV is generally considered to be the standard for achieving partial immersive experience, and 180° FOV is the standard for VR to achieve full immersion. Currently, most VR devices offer FOVs between 90° and 120°.
- EyeBox
The EyeBox defines the range within which a user’s eyes can move while still perceiving the image clearly. For a comfortable experience, the EyeBox must accommodate eye movement and variations in user eye spacing.
The entry pupil diameter of the human eye is approximately 4 mm, making this the minimum size for the EyeBox. When using VR devices, users usually move their eyes. And each person’s eye distance is different, and the condition of wearing the device is also different when using it. So the EyeBox should be as big as possible. Expanding the EyeBox improves comfort but complicates VR optical design and may impact other performance metrics.
- Distortion
VR optics transmits the virtual picture to the human eye through the optical system, and the human eye as the entrance pupil is outside the optical system, the system is asymmetric, and due to the large field of view requirements, the optical system often has more obvious distortion. Distortion occurs when the optical system transmits images with geometric inaccuracies, such as “pincushion distortion (pillow distortion),” where images stretch at the edges.
Distortion is intrinsic to VR optical systems due to their asymmetrical design and large FOV requirements. Pre-processing images into a “barrel” shape compensates for distortion but reduces resolution. Improved optical designs can alleviate processing demands and enhance quality.
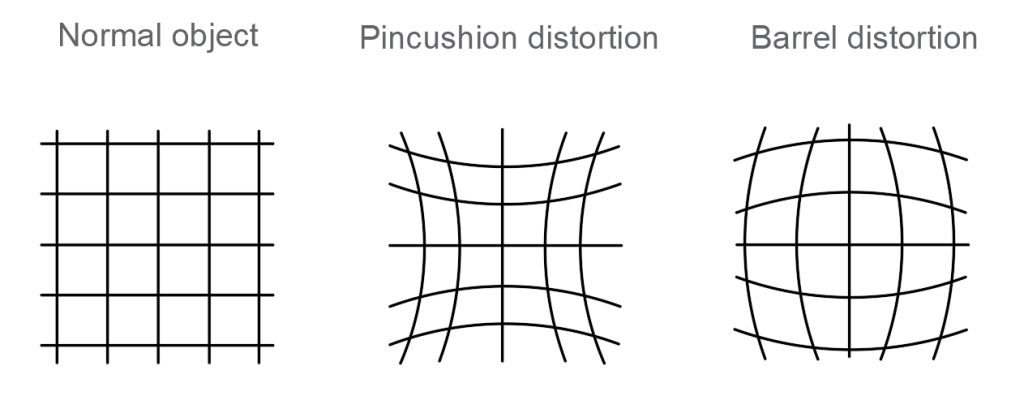
- Dispersion
Dispersion is a situation that most VR devices encounter when they are used, manifesting as color fringing, where different wavelengths of light (colors) diverge at the image’s edges. This is because the VR device transmits the signal to the human eye through refraction and transmission, so with the increase of the field of view, the vertical color difference will be more obvious.
Off-axis light beam of different wavelengths is focused through the lens after the height of the plane is different, that is, the magnification of each wavelength after imaging is different, so the vertical axis color difference is also called the power color difference. The focal points of multiple wavelengths are sequenced in the direction of the height of the image plane, and the final visible edge of the image plane produces a rainbow band.
Lens combinations with differing Abbe coefficients correct chromatic aberrations but increase cost and weight. Image pre-processing is another method to counteract dispersion.
Other key indicators in VR optics include resolution, pupil spacing, entry pupil distance, volume, and weight, all of which influence the overall design and usability of the optical system.
Optical solutions for VR devices
- Aspherical lens
In theory, near-eye displays can be achieved using a single spherical lens. However, spherical lenses suffer from significant spherical aberration, resulting in poor imaging performance. Additionally, spherical lenses exhibit large field curvature, leading to poor image quality at the edges of the field of view. Spherical lenses also have fewer adjustable parameters, limiting their ability to address these issues effectively. The surface of an aspherical lens is irregular, with curvature that changes depending on the lens’s height. By adjusting aspherical parameters, spherical aberration can be eliminated, and field curvature minimized. This is particularly effective when both lens surfaces are aspherical, offering significantly better imaging quality compared to spherical lenses.
Processing aspherical lenses is more complex than spherical lenses. While traditional precision polishing technology offers higher accuracy, it is time-consuming, expensive, and unsuitable for mass production. Injection molding is a viable alternative for manufacturing plastic aspherical lenses. In this method, heated plastic is injected into a stainless steel mold, and after a cooling period, the molded aspherical lens is removed. Post-processing, such as polishing and strengthening, can enhance the component’s finish, durability, and other properties. Injection molding is ideal for producing plastic aspherical lenses in large batches with moderate quality and low thermal stability. This method is currently the most common for VR aspherical lens solutions due to its low cost.
- Fresnel lens
Fresnel lenses are composed of a series of concentric grooves etched into plastic. These grooves are designed to minimize the amount of optical material while preserving the surface curvature. This design makes Fresnel lenses adaptable for various applications, including VR.
The advantages of Fresnel lenses include high brightness, a large field of view, and shorter working distances. By removing part of the optical material, Fresnel lenses are much thinner and lighter than aspherical lenses. Currently, most Fresnel lenses used in VR optics are manufactured using injection molding processes, enabling large-scale production and cost reductions. Fresnel lenses are the preferred solution for most current VR headsets.
However, Fresnel lenses have significant drawbacks. They suffer from substantial aberration, leading to lower image quality, especially at the edges of the field of view. VR devices equipped with Fresnel lenses also experience more distortion, often necessitating advanced pre-processing of images to improve overall quality.
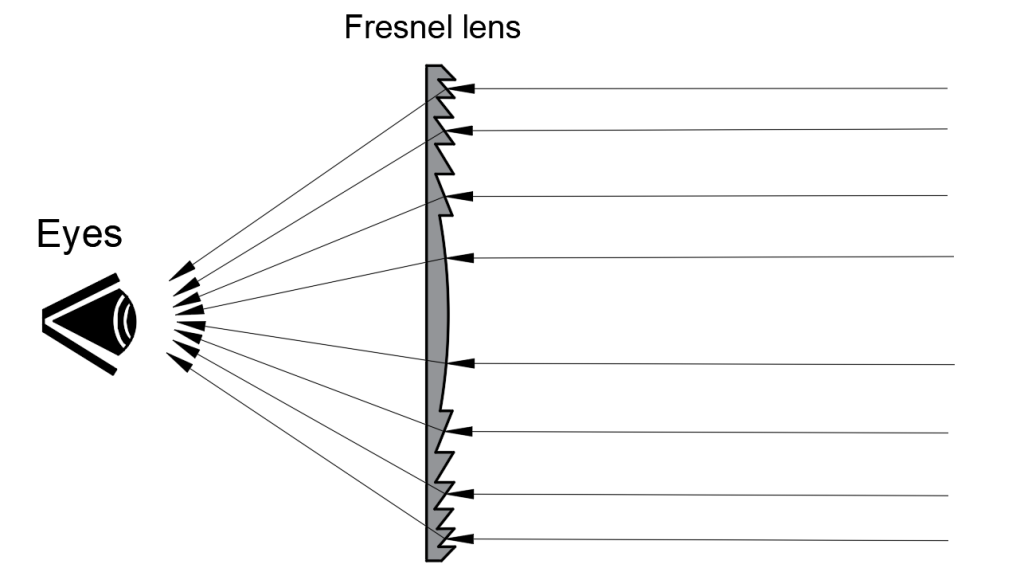
- Pancake Scheme
Although Fresnel lenses reduce the working distance and optical material required, they can still result in bulky equipment. The Pancake solution was developed to create even lighter and more compact VR devices and is currently considered one of the most promising VR solutions.
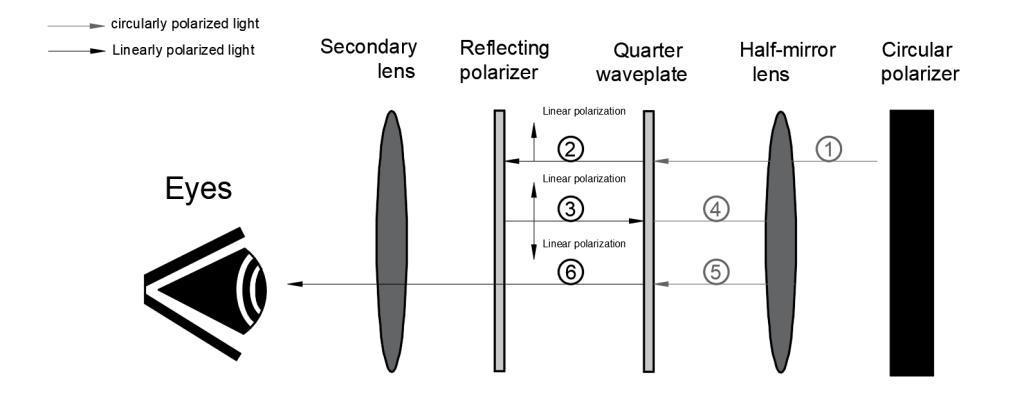
The Pancake scheme utilizes a folding optical path design, which can involve single-chip, two-chip, or multi-chip return configurations. In this design, light undergoes multiple reflections and refractions between lenses before entering the user’s eye. The Pancake solution reduces the distance between the screen and the lens, significantly shortening the overall length of the optical module. Another advantage of Pancake optics is that lens combinations enhance edge image quality, reduce distortion, and improve contrast, clarity, and sharpness.
It is worth noting that the Pancake solution requires the screen’s outgoing light to be circularly polarized. The lens surface must include a layer of PBS (polarizing beam splitter) film and a 1/4 wave plate. Circularly polarized light reflected on the lens surface is converted to linearly polarized light by the 1/4 wave plate, then reflected by the PBS film. After passing through the 1/4 wave plate again, it reverts to circularly polarized light. This circularly polarized light reflects off the semi-reflective film on the screen side, undergoes a secondary reflection, and is converted into linearly polarized light in another polarization direction upon a second pass through the 1/4 wave plate. The PBS film is highly transparent to linearly polarized light in this direction, transmitting the light to the human eye. This arrangement significantly reduces stray light.
However, the Pancake solution also has notable limitations. Due to multiple reflections and refractions, energy utilization is reduced, resulting in lower brightness. Additionally, the complex design increases production costs. While polarized light minimizes most stray light, it cannot completely eliminate it.
Optimizing VR Optics
In summary, VR optics are essential for creating immersive experiences by projecting clear, realistic images to the user’s eyes. Advances in field of view, EyeBox design, distortion control, and dispersion correction have significantly improved VR headset performance, reducing eye strain and maximizing comfort. Optical solutions such as aspherical lenses, Fresnel lenses, and the Pancake scheme offer different strengths and trade-offs regarding quality, cost, and device weight. As VR technology continues to evolve, ongoing improvements in VR lens design and material processes will be critical for achieving sharper images, broader fields of view, and lighter devices. These innovations will make VR experiences more accessible and comfortable, driving greater adoption across gaming, training, and professional applications.
Related Content